Introduction:
What is Light:
Light, or Visible Light, commonly refers to electromagnetic radiation that can be detected by the human eye.
“Darkness cannot drive out darkness: only light can do that.”
Sun: It is the natural source and is considered as the oldest source of light energy.
Electric bulbs: They are an important artificial source of light.
Flame: On burning a fuel, it will produce a flame which emits light.
Importance of Light:
Terrestrial and aquatic micro-organisms are exposed to both visible and UV radiation. In some circumstances, such as survival of enteric microorganisms in seawater, light of both visible and UV wavelengths is a major factor.
“Gentleman, It is the microbes who will have the last word,” (Loius Pasteur)
Light is, of course, essential to primary production through photosynthesis. Higher plants are adapted to use the highest intensity of light available, but the exposure of most algae and cyanobacteria to light of full intensity would lead to rapid bleaching, loss of photosynthetic ability and ultimately death
It has been hypothesized that the underlying cause is oxidative stress (Goumelon et al., 1994).
In the light, both strains of bacteria take in more organic carbon, including sugars, metabolize them faster.
In the dark, those functions are reduced, and the bacteria increase protein production and repair, making and fixing the machinery needed to grow and divide.
1. How UV Radiation affects Microbial Gwoth
Susceptibility to Photoinactivation:
Susceptibility to photo-inactivation depends both on species and individual strains, many pigments having a significant protective role.
Photosensitizers may be endogenous within the cell, while exogenous photosensitizers, such as humic substances, can also be involved
Susceptibility to UV Radiation:
Susceptibility, especially to UV radiation, is usually significantly greater in micro-organisms from human and animal sources, where exposure is minimal, than in similar micro-organisms from the external environment.
This may be demonstrated by reference to Leptospira, the free-living L. biflexa being far more resistant than the parasite L. interrogans.
Resistance to UV radiation is, however, highly conserved in subsurface microorganisms, which are effectively shielded.
Sensitivity to UV-B (280-315 nm) is greatest and populations can be under stress even at normal levels.
Increased UV-B irradiation, for example resulting from the thinning of the stratospheric ozone layer, has decreased primary production by phytoplankton in Antarctica by 6–12%. Decreases in primary production have been noted elsewhere.
Sunscreen Compounds:
Number of strategies have been developed amongst these micro organisms to avoid harmful effects while gaining greatest benefit from exposure to light.
Some, for example, produce ‘sunscreen’ compounds which absorb UV-B and thus reduce the likelihood of damage to target cellular compounds.
Strategies for High Light Intensity:
An alternative strategy involves motility and a movement to the zone of optimum light intensity, where conditions permit photosynthesis without reducing growth.
An exception is the red freshwater flagellate Euglena sanguinea which contains photoprotective pigments and which orientates itself to light (Gerber and Hader, 1994).
This enables the flagellate to take advantage of high light intensities and the stress-induced lack of competition at the water surface.
No strategy for minimizing exposure to UV radiation, however, can be entirely successful in preventing a degree of damage.
UV Radiations and Damage to Microbes
The damage primarily involves DNA, which absorbs UV light, the energy so
released causing dimerization between adjacent pyrimidine residues on the same DNA strand.
The occurrence of the pyrimidine dimers triggers a repair mechanism which excises them, exposing a short region of single-stranded DNA on the opposite strand.
Single-stranded regions are also formed if a replication fork passes a pyrimidine dimer that has not been excised.
The formation of the single stranded regions induces the SOS system, a general DNA repair system.
This, in turn, induces at least nine enzymes that fill the gaps opposite the single-stranded.
The SOS system is rapid acting, but relatively inaccurate, being described as error-prone repair.
This accounts for the mutations common after exposure to UV and, of course, the deliberate use of UV as a mutagen in genetic studies.
A second repair system involves excising defective sections of a DNA strand and their replacement by insertion of new nucleotides.
This system is relatively error free. The relative importance of the two systems varies in different bacteria.
Bacteria also differ in overall susceptibility to UV damage. Radiation-resistant bacteria, such as Deinococcus, for example, have highly effective DNA repair mechanisms, although it is also possible that the DNA of radiation-resistant bacteria is inherently more stable than that of the less resistant..
Research has shown, however, that negative responses to UV irradiation are not necessarily due to exposure to UV-B alone but that interactions with the effects of UV-A (320–400 nm) and ‘photosynthetic active radiation’ (400–700 nm) are also of major importance (Nielsen and Eklund, 1995).
How Visible Light(Red and Blue) affect Microbial Growth:
Visible light (400-800 nm) at high intensity was previously found to kill bacteria that are frequently found in infected wounds, while low-power white light enhances bacterial proliferation.
The phototoxic effect was found to involve induction of reactive oxygen species (ROS) production by the bacteria blue (400-500 nm) light illumination was found to be higher than that of red (500-800 nm).
Within the blue range, light of 415 nm induced more ROS than 455 nm, which correlated with results obtained for the reduction in colony count of S. aureus and E. coli following illumination using equal intensities of these two wavelengths.
Intense blue light, preferably at 415 nm, could be used for bacterial eradication.
Low intensity of visible light can be dangerous since it may promote proliferation of the microorganisms
Visible light at high intensity was found to kill bacteria while low-power light in the visible and near infrared region enhances bacterial proliferation.
Intense blue light, preferably at 415nm, is better than red light for bacteria killing. Red light kills bacteria via photodynamic action
Blue light (470 nm) effectively inhibits bacterial (Leuconostoc mesenteroides, Bacillus atrophaeus and Pseudomonas aeruginosa) and fungal growth e filamentous fungi, Penicillium digitatum and Fusarium graminearum when exposed to pure blue light only plus a photosensitizer.
How Infrared radiations affect Microbial Growth:
Treatment of microorganisms via IR heating results in breakage of cell walls, cytoplasmic content leakage, cytoplasm shrinkage and degradation in mesosome, and damage to the cytoplasm as evidenced by transmission electron microscopy .
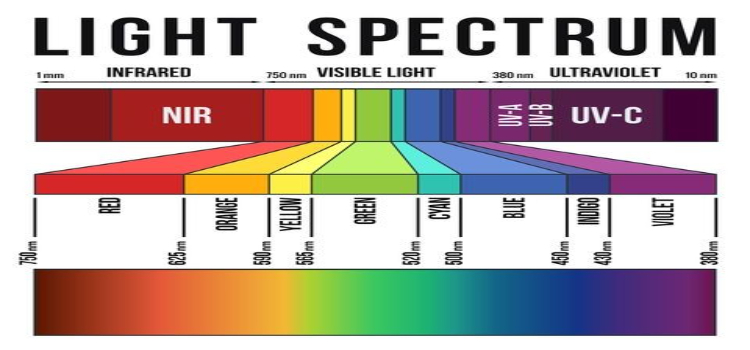
Increase in the power of the IR heating source increases the temperature and total energy absorbed by microorganisms, leading to microbial inactivation in a short time
Some kinds of bacteria, such as Escherichia coli, change these molecules into negatively charged ions. … When zapped with near-infrared light, the ions absorb the light and heat their surroundings, killing the bacteria.
Transient exposure of FIR induced compositional and temporal changes in gut microbiota of mice.
FIR (far infrared) exposure stimulated growth of the gut beneficial bacteria.
FIR exposure promoted growth of the gut SCFAs-producing bacteria.
Conclusion:
Light is, of course, essential to primary production through photosynthesis.In Case of Microbes Different type of lights has different impact on the growth of microbes.
In Visible light Blue light has lethal effect on microbes as compared to red light. Intense blue light, preferably at 415 nm, could be used for bacterial eradication.
UV The damage primarily involves DNA, which absorbs UV light, the energy so released causing dimerization between adjacent pyrimidine residues on the same DNA strand.
Treatment of microorganisms via IR heating results in breakage of cell walls, cytoplasmic content leakage, cytoplasm shrinkage and degradation in mesosome, and damage to the cytoplasm as evidenced by transmission electron microscopy